Altitude Training Simulation Through HIIT: Oxygen Restriction Methods and Benefits
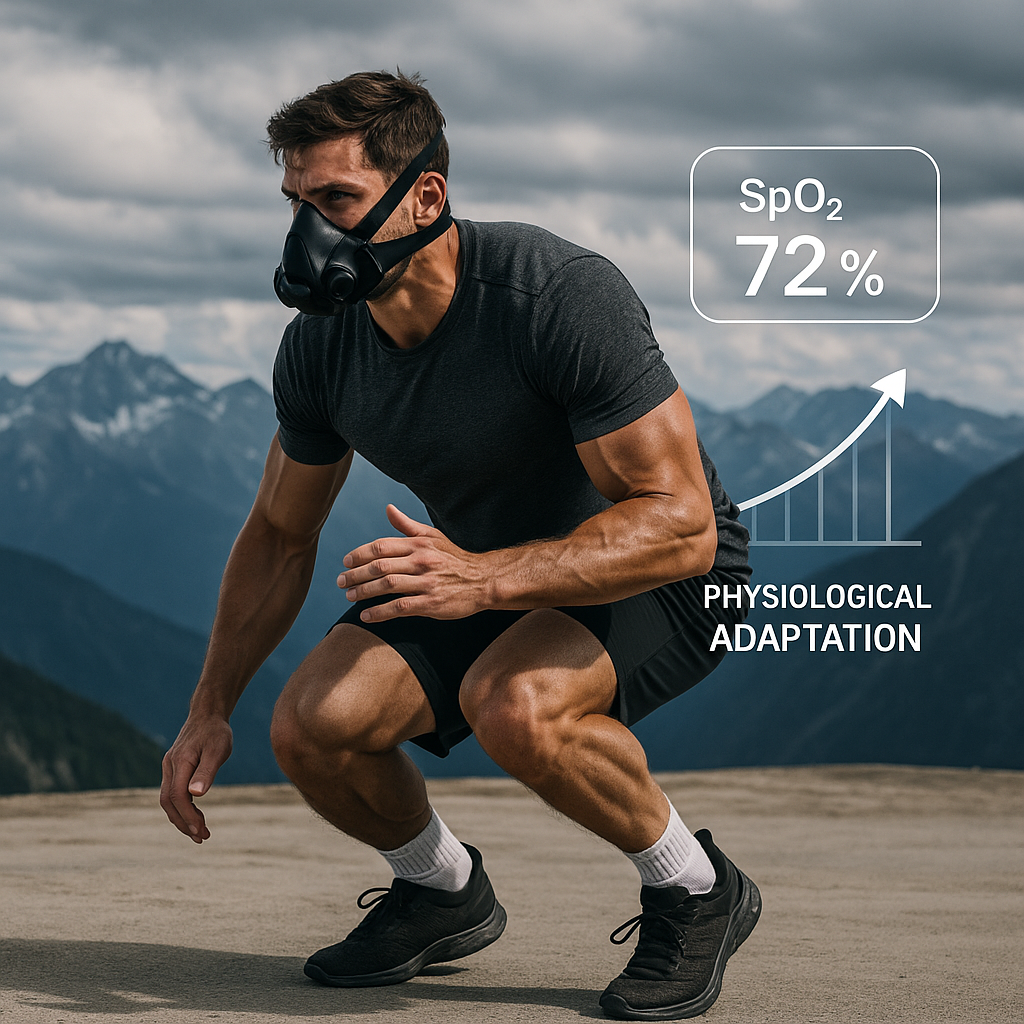
The Altitude Advantage: Performance Adaptations at Elevation
Elite endurance athletes have long recognized the powerful performance benefits of training at altitude. The reduced oxygen availability at elevations above 5,000 feet (≈1,500 meters) creates a hypoxic environment that triggers a cascade of physiological adaptations designed to improve oxygen delivery and utilization. These adaptations include increased red blood cell production, enhanced mitochondrial density, improved capillarization, and optimized metabolic efficiency—all contributing to substantial performance improvements when returning to sea level.
However, traditional altitude training presents significant challenges, including geographic limitations, financial costs, logistical complexities, and the individual variability in adaptation responses that might compromise training quality. These barriers have driven researchers and coaches to develop methods for simulating altitude's physiological effects without actually ascending to elevation. Among the most promising approaches is the strategic implementation of specialized high-intensity interval training protocols that create similar hypoxic stimuli through intense metabolic demands and specific breathing manipulations.
This comprehensive guide explores the science behind altitude training adaptations and provides practical protocols for simulating these effects through strategically designed HIIT methodologies accessible to athletes and fitness enthusiasts regardless of geographic location. By understanding the underlying mechanisms and implementing these specialized approaches, you can potentially access many of the same performance benefits traditionally restricted to those with access to high-altitude training environments.
The Physiological Impact of Altitude Exposure
To effectively simulate altitude training effects, it's essential to first understand the specific physiological challenges and adaptations created by natural elevation exposure. At higher altitudes, the partial pressure of oxygen decreases substantially despite the air still containing approximately 21% oxygen—the same as at sea level. This reduced pressure makes it more difficult for oxygen to bind to hemoglobin in the bloodstream, creating a state of arterial hypoxemia (reduced blood oxygen content) that triggers a series of acute and chronic adaptations.
The primary acute responses to altitude exposure include increased ventilation (breathing rate and depth), elevated heart rate at submaximal intensities, and reduced plasma volume that temporarily concentrates red blood cells. These immediate changes represent the body's attempt to compensate for reduced oxygen availability by increasing oxygen intake and circulation. However, these initial responses don't improve performance and actually typically reduce exercise capacity during the early adaptation phase by approximately 1-2% for each 1,000 feet of elevation gain above 5,000 feet.
The valuable chronic adaptations begin developing after several days of continuous exposure and include several key changes. Erythropoietin (EPO) production increases significantly, stimulating red blood cell production to enhance oxygen-carrying capacity. Research shows that three weeks at moderate altitude (6,500-8,000 feet) typically increases total hemoglobin mass by approximately 5-9% in responsive individuals. Capillary density increases in working muscles, reducing the diffusion distance for oxygen delivery to mitochondria. This adaptation typically shows increases of 10-15% following 3-4 weeks of altitude exposure. Mitochondrial density and efficiency improves, with studies showing 5-15% increases in oxidative enzymes that enhance cellular energy production capacity. These combined changes enhance overall oxygen utilization capacity, potentially improving sea-level performance by approximately 1-3% for endurance activities—a substantial margin in competitive athletics.
The Limitations of Traditional Altitude Training
Despite its potential benefits, traditional altitude training presents significant practical challenges that limit accessibility and effectiveness for many athletes. The geographic limitation represents the most obvious barrier—athletes must relocate to areas with appropriate elevation, often requiring significant travel, expense, and time away from normal training environments and support systems. Suitable training facilities might be limited at altitude locations, compromising workout quality and specificity. Financial considerations present substantial obstacles for non-elite athletes, with costs including travel, accommodation, and potentially lost income during extended altitude camps.
Beyond these practical challenges, physiological limitations also affect traditional altitude training effectiveness. The "Live High, Train Low" model has emerged as the gold standard approach, where athletes sleep at moderate altitude (6,500-8,000 feet) to stimulate adaptations but train at lower elevations to maintain training quality and intensity. However, this model requires specific geographic settings where such elevation differences are easily accessible—primarily places like Flagstaff/Phoenix, Arizona or similar mountain/valley combinations. Without this optimal setup, training quality often suffers significantly, with research showing approximately 5-10% reduction in sustainable workout intensity at moderate altitudes compared to sea level. This intensity reduction can potentially compromise training adaptations despite the altitude stimulus.
Individual response variability represents another significant limitation, with research indicating approximately 30-40% of individuals qualify as "non-responders" to altitude training, showing minimal hemoglobin mass increases despite proper implementation. These individual differences appear partially genetically determined and difficult to predict without prior altitude exposure. Additionally, illness risk increases at altitude, with studies showing approximately 30% higher upper respiratory infection rates during altitude camps, likely due to the drying effects of increased ventilation and potential immune system suppression from the physiological stress of adaptation. These limitations have driven exploration of alternative methods for creating similar adaptive stimuli without the associated challenges of geographic relocation.
HIIT as an Altitude Simulation Strategy
High-intensity interval training offers unique mechanisms for creating hypoxic stimuli similar to those experienced at altitude, particularly when implemented with specific protocol structures and breathing techniques.
The Physiological Parallels Between HIIT and Altitude
The metabolic demands of properly structured HIIT create localized muscle hypoxia that parallels certain aspects of altitude exposure despite occurring through different primary mechanisms. During high-intensity intervals, oxygen demand in working muscles can exceed supply by 10-15 fold compared to resting requirements. This creates a situation where muscles operate in a functionally hypoxic environment even with normal atmospheric oxygen availability. Blood oxygen saturation typically drops to 91-94% during very high-intensity intervals (compared to normal 97-99%), approaching values seen at moderate altitudes of approximately 4,000-6,000 feet. This reduction stems primarily from the muscles extracting oxygen from blood faster than the cardiorespiratory system can resupply it during maximum-intensity efforts.
The repeated hypoxic-normoxic cycles created during interval training (hypoxia during work intervals followed by reoxygenation during recovery) potentially create even more powerful adaptive stimuli than continuous hypoxia. Research indicates that this intermittent hypoxic pattern upregulates hypoxia-inducible factor 1-alpha (HIF-1α)—a key signaling protein that mediates many beneficial altitude adaptations—more substantially than continuous moderate hypoxia. Studies show approximately 2-3 fold greater acute HIF-1α activation following high-intensity intervals compared to moderate continuous exercise, explaining why properly structured HIIT may simulate certain altitude adaptations more effectively than continuous training even in hypoxic environments.
The muscle fiber recruitment patterns during HIIT further enhance the altitude simulation effect. Maximum-intensity intervals necessarily recruit high-threshold Type II muscle fibers that have lower capillary density and mitochondrial content than Type I fibers. These fibers experience more severe oxygen limitation during high-intensity work, creating powerful localized hypoxic stimuli that trigger compensatory adaptations including enhanced capillarization and mitochondrial development specifically in these otherwise less oxidatively efficient fibers. This targeted development significantly enhances overall oxygen utilization capacity, similar to adaptations observed following altitude training but achieved through a different physiological pathway.
Breath Manipulation Techniques to Enhance Hypoxic Stimulus
Beyond the inherent hypoxic stimulus created by high-intensity exercise, specific breathing techniques can further enhance the altitude simulation effect during HIIT. These approaches manipulate breathing patterns to create additional hypoxic stress that amplifies the adaptive stimulus beyond what exercise intensity alone produces. When properly implemented, these techniques can significantly augment the altitude-like adaptations without requiring specialized equipment or facilities.
Restricted breathing volume during recovery intervals represents one effective approach. This technique involves deliberately taking shallower breaths during recovery periods while maintaining normal breathing frequency. Research indicates that maintaining breathing volume at approximately 60-70% of what would naturally occur during these periods reduces blood oxygen saturation by an additional 3-5% beyond the reduction from exercise alone. This approach simulates the hypoventilation that naturally occurs at altitude due to lower atmospheric oxygen pressure. The implementation typically involves focusing on shallow chest breathing rather than deep diaphragmatic breathing during recovery intervals, creating a sustained hypoxic stimulus throughout the entire HIIT session rather than only during work intervals.
Nasal-only breathing during moderate-intensity intervals provides another effective approach for enhancing hypoxic stimulus. Breathing exclusively through the nose during work intervals of moderate intensity (approximately 80-85% of maximum heart rate) reduces maximum ventilation capacity by approximately 30-40% compared to combined mouth and nasal breathing. This restriction creates a moderate hypoxic stimulus as oxygen demand exceeds the supply capabilities of nasal-only breathing. Studies show blood oxygen saturation typically drops to 90-92% with this approach during intervals that would maintain 94-96% saturation with unrestricted breathing. This technique works most effectively with moderate-intensity intervals slightly below maximum effort, as truly maximum intensities become impossible to sustain with nasal-only breathing.
Extended exhale breathing patterns offer a third approach for enhancing hypoxic stimulus during HIIT. This technique involves extending the exhale phase of breathing to approximately twice the duration of the inhale phase (example: 2-second inhale followed by 4-second exhale). Research indicates this breathing pattern reduces average oxygen uptake by approximately 5-8% during equivalent workloads compared to spontaneous breathing patterns. The extended exhale creates a longer period where fresh oxygen isn't being inhaled while carbon dioxide continues accumulating, creating mild hypercapnia (elevated CO2) alongside the hypoxic stimulus. This combined stimulus appears particularly effective for triggering certain altitude-like adaptations, especially related to ventilatory efficiency and tolerance to blood gas disturbances during high-intensity exercise.
Specialized HIIT Protocols for Altitude Simulation
Building on the physiological parallels and breathing techniques, specific HIIT protocol structures can optimize the altitude simulation effect based on the primary adaptations targeted.
The Hypertrophic Hypoxic Protocol
This protocol specifically targets erythropoietic (red blood cell production) responses similar to those seen during altitude exposure. The structure emphasizes creating substantial systemic hypoxemia (reduced blood oxygen) rather than just localized muscle hypoxia, triggering the kidney-mediated EPO release that stimulates red blood cell production. Research indicates that this systemic hypoxic stimulus requires longer intervals at very high intensity with incomplete recovery to create sufficient arterial desaturation for triggering these pathways.
The protocol structure typically involves 4-6 intervals of 2-3 minutes at approximately 90-95% of maximum heart rate (RPE 8-9/10), followed by short recovery periods of just 60-90 seconds that prevent complete reoxygenation between efforts. This shortened recovery creates a compounding oxygen debt across intervals, with blood oxygen saturation typically declining progressively through the set. Research shows oxygen saturation often reaches 88-90% during the final intervals of this protocol—comparable to saturation levels at approximately 7,000-8,000 feet of elevation. This level of desaturation appears sufficient to stimulate modest increases in erythropoietin production, with studies showing approximately 15-30% acute EPO elevation following this protocol compared to baseline levels.
Implementation considerations include selecting exercise modalities that engage large muscle masses to maximize systemic oxygen demand. Running on an incline, rowing, or skiing movements typically create the greatest systemic hypoxic stimulus due to the large muscle recruitment patterns. The restricted breathing volume technique described earlier proves particularly valuable during the brief recovery periods of this protocol, maintaining hypoxic stimulus even during these short breaks. For optimal adaptation, this protocol should be implemented 2-3 times weekly for at least 4-6 weeks, with studies showing approximately 2-4% increases in total hemoglobin mass following this timeframe of consistent implementation—approximately 30-40% of the adaptation typically seen with actual altitude exposure of similar duration.
The Mitochondrial Development Protocol
While the hypertrophic protocol focuses on blood oxygen-carrying capacity, this approach targets the peripheral adaptations to hypoxia, particularly mitochondrial density and function. These adaptations prove equally valuable for performance enhancement and often respond more rapidly than erythropoietic changes. The protocol structure emphasizes repeated exposures to severe but brief local muscle hypoxia while allowing sufficient recovery for maintaining high-quality efforts throughout the session.
The protocol structure typically involves 10-12 intervals of 60-75 seconds at maximum sustainable intensity (RPE 9/10), followed by active recovery periods of 90-120 seconds at very low intensity (RPE 2-3/10). This format creates severe local muscle hypoxia during the work intervals while allowing sufficient but not complete recovery between efforts. Research indicates this structure upregulates PGC-1α (peroxisome proliferator-activated receptor-gamma coactivator-1 alpha)—the primary regulator of mitochondrial biogenesis—by approximately 5-7 fold above baseline following a single session. This acute response, when repeated consistently, leads to increases in mitochondrial density and function that parallel adaptations seen following altitude training.
Implementation considerations for this protocol include selecting exercise modalities that create intense local muscle demands while not necessarily maximizing systemic cardiovascular strain. Modalities like cycling, skierg, or localized functional movements typically work effectively by creating intense metabolic demand in specific muscle groups. Nasal-only breathing during the work intervals can enhance the localized hypoxic stimulus, though this typically requires slightly reducing absolute exercise intensity to maintain the prescribed interval duration. For maximum adaptation, this protocol should be implemented 2-3 times weekly on non-consecutive days, with research showing approximately 15-25% increases in mitochondrial enzyme activity following 6 weeks of consistent implementation—comparable to adaptations seen following similar duration altitude exposure.
The Hypoxic Repeat Protocol
This advanced protocol combines elements of traditional interval training with specialized breathing techniques to create a more comprehensive altitude simulation effect. The structure utilizes a "hypoxic preloading" approach where deliberate hypoventilation precedes high-intensity efforts, creating a compounded hypoxic stress that potentially triggers more robust adaptive responses than either component alone. This protocol represents a more advanced approach that should only be implemented by individuals with substantial previous HIIT experience and no underlying cardiovascular or respiratory conditions.
The protocol structure involves breath-hold preparation periods followed immediately by high-intensity efforts. A typical implementation includes 8-10 sets of: 20-30 seconds of restricted breathing (holding breath at approximately 70% of maximum breath-hold capacity) while standing or walking, followed immediately by a 30-second maximum-intensity effort, then a 2-minute complete recovery period with normal breathing. The pre-effort breath restriction creates initial hypoxemia, which the subsequent high-intensity effort then compounds, creating a particularly powerful hypoxic stimulus. Research indicates this approach can reduce blood oxygen saturation to 85-88% during the work intervals—comparable to levels experienced at elevations of 9,000-11,000 feet.
Implementation considerations include prioritizing safety with this more advanced protocol. Heart rate and oxygen saturation monitoring (if available) provide valuable feedback to prevent excessive desaturation that might compromise safety. The absolute work intensity in this protocol is typically lower than standard intervals due to the compromised initial state, but the relative physiological strain remains very high. Exercise selection should emphasize modalities with limited technical requirements, as coordination may be slightly compromised during the hypoxic state. Starting with shorter breathing restriction periods (10-15 seconds) and gradually extending the duration over several sessions allows safer adaptation to this challenging protocol. For most individuals, implementing this protocol once weekly alongside other less extreme approaches provides sufficient stimulus while minimizing potential excessive stress or overtraining risk.
Practical Implementation Strategies and Considerations
Successfully implementing altitude simulation through HIIT requires thoughtful attention to various practical considerations to maximize benefits while ensuring safety and sustainable progression.
Monitoring Approaches for Optimizing Hypoxic Stimulus
Effective implementation benefits from appropriate monitoring strategies that help individualize the hypoxic stimulus based on personal responses. These approaches allow optimal adaptation by ensuring sufficient stimulus without excessive stress that might compromise safety or training quality. Both technological and subjective monitoring methods provide valuable implementation guidance.
Pulse oximetry represents perhaps the most valuable technological monitoring approach, providing real-time measurement of blood oxygen saturation (SpO2) during training. Portable fingertip pulse oximeters offer affordable and accessible options for most athletes, though accuracy during high-intensity movement may be limited. For maximum monitoring value, measure SpO2 immediately following work intervals rather than during the intervals themselves, when motion artifacts might compromise accuracy. Research suggests optimal adaptive stimulus occurs when SpO2 reaches approximately 88-92% during or immediately following intense intervals—approximately the saturation level experienced at 6,000-8,000 feet elevation. Notably, maintaining saturation below 85% for extended periods creates excessive stress with potentially diminishing returns and increased risk, suggesting this level as a reasonable lower limit for most training purposes.
Heart rate recovery metrics provide another valuable monitoring approach, particularly when direct oxygen saturation measurement isn't available. The delayed heart rate recovery typically observed at altitude due to continued respiratory compensation can be used as an indirect indicator of hypoxic stress during HIIT sessions. A practical approach involves comparing heart rate recovery at 60 seconds post-interval between normal sessions and those implementing hypoxic techniques. A relative delay in recovery of approximately 8-12 beats per minute during hypoxic sessions compared to normal breathing sessions at equivalent workloads suggests appropriate hypoxic stimulus has been achieved. This comparative approach requires establishing personal baseline recovery patterns before implementing hypoxic techniques.
Subjective monitoring provides complementary information through ratings of perceived breathing discomfort and air hunger. Using a 1-10 scale for breathing discomfort (with 10 representing maximum sustainable discomfort) helps gauge appropriate implementation intensity. Research suggests targeting ratings of 7-8/10 during work intervals with hypoxic techniques to create sufficient stimulus without excessive stress. Importantly, normal HIIT without breathing manipulation typically produces ratings of 4-6/10 on this scale, so the hypoxic techniques should noticeably increase breathing discomfort to create appropriate stimulus. This subjective approach proves particularly valuable when technological monitoring options aren't available, though it requires honest self-assessment and familiarity with the difference between productive discomfort and excessive strain.
Periodization Strategies for Altitude Simulation Training
Effectively incorporating altitude simulation protocols into a comprehensive training program requires strategic periodization that optimizes adaptations while preventing overtraining. The relatively higher physiological stress of these approaches necessitates careful integration with other training elements and appropriate progression over time. Periodization considerations include both within-week scheduling and longer-term implementation cycles.
Within-week scheduling should balance hypoxic HIIT sessions with adequate recovery and other training elements. Research suggests limiting these specialized protocols to 2-3 weekly sessions, with at least one full recovery day between sessions to allow appropriate adaptation. A typical weekly structure might include one session of the hypertrophic protocol, one session of the mitochondrial protocol, and one standard non-hypoxic quality session that allows higher absolute training intensities without the breathing limitations. This balanced approach targets multiple adaptation pathways while preventing excessive cumulative stress. For endurance athletes, scheduling these sessions following easier aerobic training days rather than after high-intensity or high-volume training optimizes adaptation capacity by ensuring fresh neuromuscular and metabolic systems for quality execution.
Block periodization approaches work particularly effectively for altitude simulation training, with 4-6 week focused blocks of consistent implementation followed by 2-3 week periods of reduced emphasis. This structure mirrors the typical pattern used in actual altitude training camps and allows sufficient exposure for meaningful adaptation while preventing potential diminishing returns or excessive physiological stress from extended implementation. During the focused implementation blocks, slightly reducing overall training volume by approximately 10-15% helps accommodate the increased physiological stress of the hypoxic sessions without compromising recovery or adaptation. The subsequent reduced-emphasis periods should maintain standard high-intensity methods while removing the specific breathing manipulations, allowing consolidation of adaptations while relieving the additional stress of the hypoxic techniques.
Annual periodization considerations should place these altitude simulation blocks strategically relative to key competitions or performance goals. Implementing a 6-week block approximately 8-12 weeks before major competitive goals allows sufficient time for adaptations to develop and stabilize while not placing the most physiologically stressful training immediately before competitions. Some research suggests implementing a shorter "booster" block of 2-3 weeks approximately 3 weeks before competition may help maximize and preserve adaptations for key events. This approach parallels the timing strategies used with actual altitude training camps, where athletes typically complete camps several weeks before major competitions rather than immediately preceding them, allowing physiological stabilization while preserving the performance benefits.
Safety Considerations and Contraindications
While altitude simulation through HIIT offers valuable benefits for many individuals, safety considerations and potential contraindications require careful attention. These specialized approaches create more substantial physiological stress than standard HIIT, making appropriate screening and implementation essential for safe and effective outcomes. Understanding both absolute contraindications and relative risk factors helps guide appropriate implementation decisions.
Absolute contraindications include several medical conditions for which these hypoxic training approaches present unacceptable risks. Individuals with uncontrolled cardiovascular conditions—including hypertension, arrhythmias, or structural heart problems—should avoid these methods due to the additional cardiac stress created by intermittent hypoxia. Respiratory conditions like moderate to severe asthma, COPD, or exercise-induced bronchospasm represent contraindications due to the potential for exacerbation during restricted breathing protocols. Pregnancy also represents a contraindication for these specialized approaches, as fetal oxygen needs require prioritization over potential maternal performance benefits. Individuals with sickle cell trait face particular risks with hypoxic training due to the increased potential for sickling events during low-oxygen conditions, making these approaches inappropriate regardless of fitness level.
Relative contraindications requiring medical clearance and careful implementation include several conditions where individualized risk assessment proves essential. Mild controlled asthma may allow modified implementation with appropriate medical supervision and medication management. Individuals with sleep apnea should approach these methods cautiously, potentially with reduced frequency and intensity, as they already experience intermittent hypoxia during sleep that may compound training-induced hypoxic stress. Previous history of exercise-related dizziness or fainting requires careful evaluation before implementing these approaches, as hypoxia potentially exacerbates these tendencies. Altitude simulation methods should be avoided during acute illness, particularly respiratory infections, regardless of severity, as the additional physiological stress may compromise recovery and potentially increase complications.
Implementation safeguards help minimize risks for appropriate candidates undertaking these approaches. Training with a partner or in supervised settings provides valuable safety oversight, particularly during initial implementation when individual responses remain unknown. Starting with more moderate protocols and gradually increasing intensity allows safer adaptation to the additional stress of hypoxic training. Proper warm-up becomes especially important before these sessions, with approximately 10-15 minutes of progressive intensity preparation helping optimize cardiovascular and respiratory readiness for the hypoxic stimulus. Maintaining adequate hydration supports optimal blood viscosity during these sessions, as dehydration compounds the potential stress of intermittent hypoxia. For those with access to appropriate technology, periodic monitoring of resting heart rate variability provides valuable insight into overall recovery status and readiness for these more demanding sessions, with declining trends suggesting the need for reduced training stress until recovery improves.
Technology and Equipment for Enhanced Altitude Simulation
While effective altitude simulation remains possible through protocol structure and breathing techniques alone, various technologies and equipment can enhance and standardize the hypoxic stimulus for potentially greater adaptation.
Respiratory Restriction Devices
Several commercially available devices provide standardized methods for creating breathing resistance that simulates aspects of altitude training. These devices typically restrict either inspiratory airflow (breathing in), expiratory airflow (breathing out), or both, creating additional respiratory muscle work and potentially enhancing the hypoxic stimulus during exercise. The standardization these devices provide potentially creates more consistent training stimulus compared to technique-only approaches, though effective implementation still requires appropriate protocol design and progression.
Inspiratory restriction masks represent the most common devices, typically featuring adjustable valves that limit airflow during inhalation while allowing normal exhalation. Research indicates these devices primarily increase respiratory muscle work rather than directly reducing blood oxygen levels when used during exercise at moderate intensities. However, when combined with high-intensity intervals, the added breathing resistance can enhance the hypoxic stimulus by approximately 2-3% additional oxygen desaturation compared to the same intervals without the device. Practical implementation typically involves starting with minimal restriction settings (typically 1,000-2,000 feet simulated elevation on adjustable devices) and gradually increasing resistance as adaptation occurs. These devices prove most valuable during the recovery periods between high-intensity intervals, where they help maintain hypoxic stimulus even as exercise intensity decreases.
Combined inspiratory-expiratory restriction devices provide more comprehensive breathing limitation that may better simulate actual altitude conditions. These systems typically use either water column resistance or spring-loaded valves to create bidirectional breathing resistance, increasing work for both inhalation and exhalation. Research indicates these devices create more substantial blood gas disturbances than inspiration-only restrictions, with approximately 3-5% greater oxygen desaturation at equivalent workloads. The expiratory restriction component appears particularly valuable for enhancing CO2 retention, which contributes significantly to the ventilatory adaptations seen with altitude exposure. Practical implementation should begin with very modest resistance settings and shorter durations (10-15 minutes) before progressively increasing as tolerance develops, as these devices create substantially greater respiratory discomfort than inspiration-only restrictions.
Optimal application of these devices involves strategic use during specific portions of training rather than continuous wear throughout all training. Research indicates that intermittent use during high-intensity intervals and their recovery periods creates more valuable adaptive stimulus than continuous use during longer moderate-intensity training. A practical approach involves using the devices specifically during the HIIT protocols described previously rather than during all training sessions. This targeted implementation maximizes the value of the additional resistance while minimizing unnecessary respiratory muscle fatigue during sessions focused on other training objectives. For most individuals, 2-3 weekly sessions using these devices provides sufficient stimulus without creating excessive respiratory muscle fatigue that might compromise overall training quality.
Intermittent Hypoxic Training Systems
More sophisticated technologies allow direct manipulation of inspired oxygen concentration independent of breathing resistance, creating standardized hypoxic environments without the need for actual altitude exposure. These systems more directly replicate the reduced oxygen partial pressure experienced at altitude, potentially creating more specific adaptive stimuli than respiratory restriction devices alone. While substantially more expensive than simple restriction devices, these systems allow precise control of the hypoxic stimulus and potentially greater overall effectiveness.
Mask-based hypoxic air delivery systems represent the most accessible option in this category. These portable systems typically use nitrogen-enrichment technology to reduce the oxygen percentage in inspired air from the normal 21% to approximately 15-16% (simulating approximately 8,000-10,000 feet elevation). The systems deliver this hypoxic air through a sealed mask worn during exercise, creating standardized hypoxic conditions independent of breathing pattern or exercise intensity. Research indicates these systems reliably reduce blood oxygen saturation to approximately 88-92% during high-intensity exercise—comparable to levels experienced at actual moderate altitude. The primary advantage over restriction devices involves the consistency of the hypoxic stimulus regardless of breathing pattern adjustments, potentially creating more reliable adaptive responses over time.
Room-based or tent-based hypoxic systems provide more comprehensive hypoxic exposure capabilities. These larger systems create hypoxic environments within enclosed spaces, allowing both exercise and potentially sleeping in hypoxic conditions. This approach better replicates actual altitude training camps, particularly the "Live High, Train Low" model when the systems are used primarily for sleeping while normal training occurs in normoxic conditions. Research indicates approximately 8-12 hours daily exposure at simulated altitudes of 8,000-10,000 feet (14-16% oxygen) creates meaningful increases in erythropoietin and subsequent red blood cell production comparable to approximately 50-60% of the response seen with actual altitude exposure. These systems require substantial financial investment but provide the most comprehensive altitude simulation capabilities outside of geographic relocation.
Implementation strategies for these technologies should follow similar guidelines to those used for actual altitude exposure, with gradual introduction and appropriate monitoring. Initial exposure should utilize higher oxygen percentages (17-18%, simulating 5,000-6,000 feet) before progressively decreasing oxygen concentration as adaptation occurs. Session duration should similarly progress gradually, starting with 20-30 minute exposures and extending based on individual tolerance and adaptation. Heart rate and oxygen saturation monitoring prove particularly valuable with these systems, as they create more substantial and consistent hypoxic stress than technique-based approaches. The consistent hypoxic stimulus these systems provide potentially allows slightly reduced frequency compared to technique-only approaches, with 2 weekly sessions potentially providing sufficient stimulus for meaningful adaptation when using these more standardized hypoxic conditions.
Combination Approaches for Maximal Benefit
For those seeking optimal altitude simulation benefits, strategic combination of multiple approaches potentially creates more comprehensive adaptation than any single method alone. These integrated approaches parallel the most effective actual altitude training models by addressing multiple physiological pathways simultaneously. While requiring greater planning and potentially financial investment, these combination strategies may provide the closest approximation to actual altitude training benefits without geographic relocation.
The intermittent hypoxic exposure plus HIIT combination offers substantial benefits with reasonable implementation complexity. This approach involves using mask-based hypoxic air delivery systems or room-based systems specifically during HIIT sessions while maintaining normal breathing conditions during other training. Research indicates this targeted approach creates approximately 15-25% greater adaptive response than either HIIT alone or hypoxic exposure during light activity, measured through changes in hemoglobin mass and maximum oxygen consumption. The hypoxic HIIT sessions essentially concentrate the adaptive stimulus during periods of already elevated metabolic stress, potentially creating synergistic signaling for adaptation. A practical implementation involves 2-3 weekly HIIT sessions using hypoxic air delivery (15-16% oxygen) during both intervals and recovery periods, creating substantial stimulus while requiring relatively limited total hypoxic exposure time.
The sleep high, train normal with hypoxic HIIT combination represents the most comprehensive altitude simulation approach. This model uses tent-based or room-based hypoxic systems during sleep (approximately 8 hours at 15-16% oxygen) combined with primarily normoxic training but with periodic hypoxic HIIT sessions. This approach closely mirrors the gold standard "Live High, Train Low" model used at actual altitude while incorporating elements of "Live High, Train High" during specific high-intensity sessions. Research indicates this combined approach potentially creates approximately 60-70% of the erythropoietic benefits of actual altitude training while maintaining higher training quality than would be possible at consistent altitude. The primary limitations involve the substantial equipment requirements and associated costs, making this approach realistic primarily for elite athletes or well-funded teams rather than recreational athletes.
Implementation periodization for these comprehensive approaches should follow similar patterns to actual altitude training camps. A typical structure involves 4-6 week focused implementation blocks with hypoxic sleeping and 2 weekly hypoxic HIIT sessions, followed by 4-6 weeks of normoxic conditions with periodic (once weekly) hypoxic HIIT sessions to maintain adaptations. This cycle can be repeated 2-3 times annually, typically with major implementation blocks occurring during base training phases rather than immediately before key competitions. This periodization allows significant cumulative adaptation while preventing potential overtraining or excessive physiological stress from continuous implementation. As with actual altitude training, individual response monitoring remains essential for optimizing protocols based on personal adaptation patterns rather than standardized recommendations alone.
Using Peak Interval for Hypoxic HIIT Protocols
The Peak Interval app provides specialized functionality that enhances the implementation of hypoxic HIIT protocols for altitude simulation.
The custom interval creation feature allows precise programming of the specialized timing structures needed for effective hypoxic training. For the hypertrophic protocol, the ability to program longer intervals (2-3 minutes) with precisely timed incomplete recovery periods (60-90 seconds) ensures the compounding oxygen debt essential for stimulating erythropoietic responses. For the mitochondrial protocol, creating consistent 60-75 second work intervals with appropriate recovery timing supports the optimal balance of hypoxic stimulus and performance quality. The breath-hold preparation periods of the hypoxic repeat protocol can be precisely programmed using the preparation timer feature, ensuring consistent implementation of this advanced technique.
The audible cuing functionality proves particularly valuable for breath manipulation techniques during intervals. Different sounds can be assigned to signal breathing pattern changes, allowing focus on exercise execution rather than timing breathing manipulations. For example, distinct tones might indicate transitions between nasal-only breathing and normal breathing, or signal when to implement restricted breathing volume during recovery periods. The programmable preparation periods between intervals support proper recovery and readiness for each subsequent high-intensity effort—particularly important during these physiologically demanding protocols.
Train at Any Altitude
Use Peak Interval's customizable timers to implement research-backed hypoxic HIIT protocols—accessing the benefits of altitude training without leaving sea level.
Download Peak IntervalPerformance Benefits and Adaptation Expectations
Understanding the realistic performance benefits and adaptation timeline of altitude simulation through HIIT helps establish appropriate expectations and implementation commitment.
Measurable Adaptation Markers
Several physiological markers provide objective feedback regarding the effectiveness of altitude simulation protocols. These measurements help verify that the implemented approaches are creating meaningful adaptation rather than simply increased training stress without corresponding benefits. Some markers require specialized testing, while others remain accessible with basic monitoring approaches available to most athletes and coaches.
Hemoglobin mass represents the gold standard measurement for erythropoietic adaptation to altitude or simulation protocols. This measurement quantifies the total circulating hemoglobin rather than just concentration, providing direct insight into oxygen-carrying capacity improvements. Research indicates well-implemented altitude simulation through HIIT typically increases total hemoglobin mass by approximately 2-4% over 6-8 weeks of consistent implementation—approximately 30-50% of the adaptation seen with actual altitude training camps of similar duration. This measurement requires specialized carbon monoxide rebreathing testing or similar methods typically available only at sports performance laboratories or advanced training centers. For those without access to such testing, trends in hematocrit and hemoglobin concentration from standard blood tests can provide partial insight, though these values are significantly influenced by hydration status and plasma volume changes independent of true hemoglobin mass changes.
Submaximal exercise heart rate provides a more accessible adaptation marker, with research showing approximately 5-8% reduction at standardized submaximal workloads following effective altitude simulation protocols. This adaptation reflects improved oxygen delivery and utilization capacity, allowing the same external work with reduced cardiovascular strain. Measuring heart rate at a fixed, moderate workload (example: 70% of maximum) periodically throughout an implementation block provides valuable feedback regarding adaptation progress. Decreasing heart rates at this standardized workload suggest effective adaptation occurring through the protocols. This measurement requires only basic heart rate monitoring capability accessible to most athletes and coaches.
Lactate response to standardized exercise offers another valuable adaptation marker. Properly implemented altitude simulation protocols typically reduce blood lactate concentration by approximately 15-25% at standardized submaximal workloads after 6-8 weeks of consistent training. This adaptation reflects improved metabolic efficiency and enhanced clearance capacity—both key benefits of effective altitude adaptation. While direct lactate measurement requires specialized equipment, the power or pace at lactate threshold provides a related measurement often available through performance testing at training centers. Improvements of approximately 3-6% in threshold power or pace typically occur following effective altitude simulation protocols, reflecting enhanced metabolic efficiency that allows higher workloads before significant lactate accumulation begins limiting performance.
Performance Transfer and Competition Benefits
The ultimate validation of altitude simulation approaches comes through actual performance improvements in target events or competitions. Understanding the likely magnitude, timeline, and context-specificity of these improvements helps establish appropriate expectations and implementation commitment for these specialized training approaches.
Endurance performance benefits typically range from approximately 1-3% following well-implemented altitude simulation protocols—a meaningful improvement in competitive contexts but less than the 2-4% often seen with optimal actual altitude training. Research indicates these performance improvements manifest primarily through enhanced sustainable intensity rather than changes in maximum capacity, with performance at around 80-90% of maximum showing greater improvement than brief, maximum efforts. The most substantial benefits typically appear in events lasting approximately 3-30 minutes, where the enhanced oxygen utilization capacity most directly influences performance outcomes. Events shorter than 3 minutes typically show more modest benefits, as anaerobic metabolism plays a more dominant role in these activities and sees less direct enhancement from the hypoxic adaptations.
The time course of performance improvement follows a relatively predictable pattern. Initial implementation typically creates a temporary performance decrement of approximately 1-2% during the first 2-3 weeks as the body manages the additional physiological stress without fully developed adaptations. Meaningful performance improvements typically emerge after approximately 4-6 weeks of consistent implementation, with peak benefits usually occurring approximately 2-3 weeks after completing a focused implementation block. This delayed peak phenomenon mirrors that seen with actual altitude training, where the best performances often occur several weeks after returning to sea level rather than immediately upon return. Understanding this timeline helps prevent premature abandonment of protocols during the initial challenging adaptation phase while appropriately timing implementation relative to key competitions.
Performance benefits preservation depends significantly on continued training quality after completing focused altitude simulation blocks. Research indicates most adaptations remain largely intact for approximately 3-4 weeks following protocol completion if normal high-quality training continues during this period. Implementing periodic "maintenance" sessions (typically once weekly) using the hypoxic techniques extends this benefit retention to approximately 6-8 weeks. This extended retention makes properly timed altitude simulation particularly valuable before competitive seasons that span several weeks, as the benefits can influence multiple competitions rather than requiring continuous implementation. For year-round benefit, most research suggests implementing 2-3 focused blocks annually with maintenance sessions between blocks provides optimal adaptation while preventing excessive accumulated physiological stress.
Individual Response Considerations
As with actual altitude training, individual response variability significantly influences outcomes from simulation protocols. Understanding these individual differences helps optimize protocol selection and implementation rather than applying standardized approaches that might not match individual response patterns. Several factors influence this response variability and can guide more personalized implementation strategies.
Genetic factors significantly influence both actual altitude and simulation protocol responses. Research indicates approximately 20-30% of individuals qualify as "high responders" showing enhanced adaptive responses to hypoxic stimuli, while approximately 30% demonstrate minimal erythropoietic response despite proper implementation. This response variation appears significantly linked to variations in the hypoxia-inducible factor (HIF) pathway genes that regulate many altitude adaptations. While genetic testing for these specific factors remains limited in commercial availability, previous response to altitude exposure provides valuable insight into likely simulation protocol responses. Individuals who demonstrated positive responses to previous altitude exposure typically show similarly enhanced responses to simulation protocols, while previous "non-responders" to altitude often show similarly blunted responses to simulation approaches.
Pre-existing fitness levels and training history also influence response magnitude. Well-trained athletes typically demonstrate more modest percentage improvements than less-trained individuals, though the absolute adaptation may be similar. This pattern reflects the smaller relative gap between current capacity and physiological potential in highly-trained individuals. However, these smaller percentage improvements often represent more meaningful competitive advantages at higher performance levels, where tiny margins frequently determine outcomes. Training history specifically with high-intensity methods also influences response patterns, with individuals regularly implementing standard HIIT typically showing more modest additional benefits from hypoxic variants compared to those for whom high-intensity training represents a novel stimulus independent of the hypoxic component.
Implementation patterns significantly affect individual responses, with consistency proving more important than occasional high-intensity sessions regardless of protocol sophistication. Research indicates that 2-3 weekly sessions consistently implemented over 6-8 weeks creates substantially greater adaptation than more intensive approaches with less consistency. This pattern highlights the importance of sustainable implementation rather than excessive early intensity that might compromise adherence. For individuals with limited training time, focusing on consistent implementation of fewer weekly sessions generally provides better outcomes than attempting higher frequencies with inconsistent execution. This individualized approach to frequency and progression based on personal schedule constraints and recovery capacity ultimately creates more meaningful adaptation than attempting to follow standardized protocols that might not match individual circumstances.
Conclusion: Bringing the Mountain to the Athlete
Altitude simulation through specialized HIIT protocols represents a scientifically supported approach for accessing many benefits traditionally restricted to those with geographic access to appropriate elevation. While these approaches may not fully replicate all aspects of actual altitude exposure, the strategic implementation of appropriate protocols, breathing techniques, and potentially supportive equipment creates meaningful physiological adaptations with practical performance benefits.
The most effective implementation typically combines multiple approaches—specialized interval structures, breathing manipulation techniques, and potentially supportive equipment—rather than relying on any single method alone. This integrated approach addresses multiple physiological pathways simultaneously, creating more comprehensive adaptation than isolated interventions. The strategic periodization of these methods into focused implementation blocks with appropriate maintenance periods between intensives provides sustainable year-round benefits while preventing excessive accumulated physiological stress.
For athletes without geographic or financial access to traditional altitude training, these simulation approaches offer a valuable alternative that democratizes many benefits previously available primarily to elite competitors. The accessibility of these methods—many requiring no specialized equipment beyond appropriate protocol knowledge—allows athletes at all levels to incorporate aspects of altitude benefit into their training regardless of location or resources. While the adaptations may prove somewhat smaller in magnitude than optimal actual altitude exposure, the performance improvements remain meaningful in competitive contexts and potentially represent the difference between podium positions or personal bests versus missed opportunities.
As research in this field continues advancing, increasingly refined protocols and implementation strategies will likely emerge, further enhancing the effectiveness of altitude simulation through HIIT. However, the fundamental principles outlined in this guide—creating appropriate hypoxic stimuli through intensity manipulation, breathing techniques, and strategic timing—will remain valuable regardless of specific protocol evolutions. By understanding these underlying mechanisms and implementing appropriate approaches based on individual circumstances and responses, you can effectively bring aspects of the mountain's benefits to your training regardless of geographic elevation.